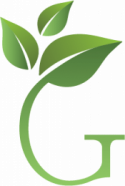
Protected: Spencer Schuchman
- Graduate Student
- SIU Carbondale
- Gage Lab
- spencer.schuchman98@gmail.com
To have your picture and bio updated please fill out this form.
To have your picture and bio updated please fill out this form.
Without question, if you must manage a herbicide-resistant weed or weeds, it will require more time and money. With fewer effective herbicide options, you will likely need to use additional herbicides in your tank mixes or make more trips over the field to control the resistant biotypes. One of the most common recommendations for preventing selection of herbicide-resistant weeds is to add a second or even third effective mode of action to the spray program. This can prevent or delay selecting for resistant biotypes and is usually necessary after resistant weeds become established. However, rotating to another herbicide mode of action to control an already resistant population can lead to additional resistance. You will need to integrate more non-chemical control tactics such as mechanical control, changing cultural practices and using other strategies such as cover crops and harvest weed seed control. This multi-pronged approach is known as integrated weed management (Figure 5). If you are not successful, weed control will be inadequate and you could lose crop yield and quality.
Currently, managing a herbicide-resistant weed population may require use of a less effective herbicide, treating weeds when they are smaller, and ensuring the herbicide is applied at the right time. According to 2022-23 estimates of custom ag operator rates from university Extension publications in Illinois, Iowa, Ohio, Maryland, and New York, each spray pass (not including the individual cost of herbicides) can cost between $5 and $15 per acre for application. Custom rates for primary tillage average $17 per acre, while custom rates for row cultivation average almost $15 per acre across the Central and Eastern Corn Belt. Depending on the resistant target weed, a study conducted in Ontario, Canada showed that glyphosate-resistant weeds increased herbicide costs on average by $38 per acre for corn and $27 per acre for soybeans. The study reported that glyphosate-resistant weeds cost the province $28 million more annually in management, while causing $15 million in yield loss.
Figure 5. Various integrated weed management (IWM) tactics are employed to manage herbicide-resistant weeds. They can include using several types of preventive tactics, such as crop rotation, tillage, hand removal, integrating cover crops, using alternative herbicides, and adopting various harvest weed seed control tactics. (Photo credits: Claudio Rubione, GROW).
For many years, weed scientists studied how many weeds it takes to impact crop yield, a concept known as “weed-crop competition.” They developed economic thresholds for individual species and the overall weed community. An economic threshold for weeds is defined as the weed population at which the cost of control is equal to the value of crop yield attributable to that control. In other words, that’s the point when the yield you would lose costs as much as the control option you would use. The action threshold is the point in time at which some control action is initiated and generally occurs just prior to reaching the economic threshold. This usually translates into the time to apply herbicides or other control measures.rnrnThese concepts were delivered by scientists to ag professionals and farmers for more than 20 years, but they were never widely adopted in the U.S. This was mostly because of the complexity and variability in developing reliable thresholds for multiple weed species at multiple locations, and the belief by many that “the only good weed is a dead weed.” With the evolution of herbicide resistance, many growers have adopted the no-seed or zero-threshold concept, which aims to control all weeds to prevent the production of weed seeds returning to the soil to create greater problems in the future. For problematic herbicide-resistant species like Palmer amaranth, a no-seed threshold is a worthy goal. However, for weeds that are easier to manage in more diverse cropping systems and where there is less concern for resistance, such as giant foxtail and common lambsquarters, the economic threshold concept still holds true.
To develop an IWM strategy on your farm, consider using the GROW Weed Management Planner to help identify useful strategies for your weed spectrum.
The Weed Management Planner was developed for the Mid-Atlantic region, but has relevance for growers across other parts of the U.S. Corn and Soybean Belt. Additional versions that focus on other regions and cropping systems should be available in the future. The current tool will help you identify common crop management and weed control strategies that can have a positive effect in managing your biggest weed problems, as well as those that could worsen certain weed infestations.
Here is an example scenario developed using the GROW Weed Management Planner.
A hypothetical farm is located in the Southern Corn Belt and grows corn and soybean in rotation, using no-till practices. The farm has problems with glyphosate- and ALS-resistant (Group 2) horseweed (marestail) and increasing problems with glyphosate- and ALS-resistant waterhemp. Additional (non-herbicide-resistant) weeds include common lambsquarters, common ragweed, and giant foxtail.
B. Effective mechanical control tactics:
C. Effective chemical tactics:
It is difficult to select multiple effective herbicide MOA for every weed species present in a field. However, this tactic is highly recommended for known resistant biotypes and weeds that have high potential to evolve herbicide resistance. In this example, herbicide-resistant horseweed and waterhemp are the driver weeds. However, when choosing the herbicide programs, be sure to also consider the other weed species present. Selected herbicides below are based on the 2024 Mid-Atlantic Weed Control Guide. Review state or regional weed management guides and a current herbicide label for detailed herbicide use recommendations.
For glyphosate- and ALS-resistant (Group 2) horseweed, use an effective burndown program with two or more effective herbicide modes of action and also include effective residuals. Example burndown herbicides for soybean include 2,4-D, dicamba (dicamba-resistant soybeans only), halauxifen (Elevore) (Group 4), and saflufenacil (Group 14). Effective residuals for waterhemp include metribuzin (Group 5), sulfentrazone and flumioxazin (Group 14) and S-metolachlor (Group 15). A number of products are available that contain these single and multiple active ingredients. For corn, burndown herbicides include 2,4-D and dicamba alone or in combination, usually mixed with a residual herbicide such as atrazine or simazine, isoxaflutole, S-metolachlor, mesotrione, or other premixes containing these plus other active ingredients.
For glyphosate- and ALS-resistant waterhemp, use effective residual herbicides at planting and timely postemergence applications that include effective residuals.
In soybean, effective residual products can include S-metolachlor and pyroxasulfone (Group 15), metribuzin (Group 5), and fomesafen, sulfentrazone, and flumioxazin (Group 14). A number of products are available that contain these single and multiple active ingredients and some of these can be applied postemergence. Effective postemergence herbicides for the resistant waterhemp in this example include 2,4-D (Group 4 for Enlist soybean), glufosinate (Group 10 for Enlist, XtendFlex, and LibertyLink soybean), and lactofen, fomesafen, and acifluorfen (Group 14).
For corn, effective residual herbicides for the resistant waterhemp in this example include atrazine and simazine (Group 5), S-metolachlor and pyroxasulfone (Group 15), acetochlor/atrazine mixtures and S-metolachlor/atrazine mixtures (Group 15/5) that can include mesotrione and bicyclopyrone (Group 27) and other active ingredients. Effective postemergence herbicides in corn include atrazine (Group 5), and mesotrione, topramezone, tembotrione, and tolpyralate (Group 27), glufosinate (Group 10) and dicamba (Group 4) containing products.
Developing an IWM Strategy – bringing it home. In this Southern Corn Belt scenario, several of these IWM tactics can have a dramatic impact on herbicide-resistant weed management.
For example, let’s say that by owning or borrowing a grain drill, the farm incorporates a rye cover crop after corn harvest, after learning the benefits obtained by cover crop adoption, including suppression of two important herbicide-resistant weeds. At soybean planting time, the farm modifies/improves the soybean planter to successfully plant green into the cover crop, which allows the cereal rye to achieve more biomass and thus weed suppression. With a second planter or one with split rows, the farmer narrows the soybean row spacing to 15 inches to help further suppress weeds and carefully chooses the herbicide program based on multiple effective modes of action.
After soybean harvest, the farmer plants a winter wheat cash crop using the grain drill to help diversify the crop rotation, which has many benefits. Now with both soybean and wheat, the farm could consider adopting harvest weed seed control (HWSC), by either modifying the combine for chaff lining or adding a seed impact mill to the combine. This would help reduce the waterhemp and other summer annual weed seedbank in soybean as well as some winter annual weeds in wheat. In addition, after wheat harvest, the farmer could seed another cover crop in late summer, such as hairy vetch plus a small grain to help suppress winter annual weeds (such as horseweed) and also provide additional nitrogen for the subsequent no-till corn crop.
Alternatively, the farmer could seed a perennial forage crop like alfalfa after wheat harvest adding yet another crop to the rotation and a very powerful weed control tactic that uses repeated mowing. Additional equipment, labor, and markets will be required for this type of big change, so consider options carefully. Finally, the farmer selects the rotational corn crop herbicide program based on multiple effective modes of action as well as other herbicide selection factors. All these IWM tactics are possible, and others could also be considered. So, what weed management strategies best fit your farm operation and need for better weed control? Please share your opinion with us here.
A number of new technologies are under development that fit into the IWM toolbox of prevention, cultural, chemical, mechanical, and biological tools, along with innovations in plant genetics and plant breeding, precision agriculture equipment and machine learning technology, information technology including the development of new decision support tools, and federal, state and private third party incentives that promote diversity in agriculture (Figure 13). Finding new cost-effective tools for agronomic weed control are challenging, but many private companies and universities around the world are actively looking for methods to help farmers manage weeds.
In addition, what can we expect from new herbicide MOA or other chemical-based strategies? Some of the major herbicide manufacturers have increased efforts to discover new herbicide molecules. Both private and public institutions are looking for naturally occurring or novel compounds that could be developed into effective commercial herbicides. Companies identify critical new enzymes and search for molecules that inhibit them, thus targeting enzymes that have not been targeted previously. See Section 10 in “The Basics of Herbicide Resistance” for more details.
1. Advances in genetics and plant breeding will likely change the way we manage pests. That includes genetic engineering and gene drives (a tool that forces certain genes to be in a species’ population that could impact their success and survival). It also encompasses the “general omics” – various disciplines in biology (including genomics, proteomics, metabolomics, transcriptomics) that impact the structure, function, and dynamics of organisms. Advances could also improve crop competition and better understand light quality interactions and plant response. Certainly better cover crops could be part of this with a focus on adding regionally adapted species and cultivars, as well as mixtures of species that perform needed functions such as winter-hardy cover crops, species with enhanced allelopathy and weed suppression, and perhaps cover crops developed by plant breeders for other desirable traits.
2. New precision ag equipment and machine learning technology is rapidly advancing in agriculture. This includes precision ag technologies such as weed detection technology, robotics, and AI-driven applications.These advancements can be applied to mechanical and chemical weed control technology. Learn more about this field on GROW’s Precision Weed Management page.
3. Don’t forget biocontrol. This area continues to advance, particularly for invasive species management in rangeland and natural areas, but also in crop production. New microbial bioherbicides, predatory insects and plant pathogens, and other new discoveries are constantly being sought and tested to help manage weeds and other pests where other control tactics are limited.
4. Though not a “new” tool, information technology or new ways to communicate and educate are rapidly advancing. Computer-driven applications such as social media platforms deliver information to far-flung audiences from multiple sources rapidly every day. Decision Support Tools are being developed to help better manage problem weeds like Palmer amaranth. This trend will likely only intensify with advancements in artificial intelligence and other information processing technology.
5. Finally, federal, state and private third party incentives that promote diversity in agriculture could help with IWM efforts. These include incentives encouraging cover crop adoption, promotion of more diverse crop rotations, novel equipment adoption, area-wide pest management implementation, and other tactics to increase cropping system diversity and reduce reliance on herbicides. This should come from both public and private sectors focused on U.S. agriculture.
Herbicide-resistant weeds spread or invade new areas just as non-resistant weeds do. The mechanisms of new weed invasion and spread fall into two main categories:
Mother Nature and Weed Biology. This includes spread by wind, water, and wildlife, and some weed species have specialized traits that help their seeds disperse naturally by these methods (Figure 5). These specialized traits can include a feathery pappus attached to each seed giving flight (horseweed and dandelion are examples), and seeds covered in barbs or burs that easily attach to the fur of animals (examples include common cocklebur and common burdock). Other examples include seeds that can pass through animals intact and potentially remain viable (includes many different species), and finally the tumbleweeds like kochia and Russian thistle that when mature break free traveling end over end spreading their mature seeds.
Human Activities. These include moving weed seeds or other plant parts with farm inputs like crop seeds, soil, manure, and compost, as well as using farm equipment or transporting freight that may be contaminated with herbicide-resistant weed seed. Using certified crop seed, regularly cleaning farm equipment, and properly composting manure and other organic waste products helps avoid moving weed seeds with these activities.
Figure 5. Seeds dispersing and/or spreading via natural processes and human activities.
To learn more about how herbicide resistant weeds spread, visit this GROW webpage.
Evolution of and selection for herbicide resistance. Herbicide-resistant individuals may be present in a weed population initially at exceptionally low frequency. With repeated application of the same herbicide or herbicide group over time, the resistant weed biotype(s) become more prominent. As an example, the widespread adoption of Roundup Ready corn, soybean, cotton, and sugarbeet allow frequent applications of glyphosate. If these crops are grown in rotation, glyphosate may be applied every season, and sometimes multiple times within a season. In addition, glyphosate is commonly used to control weeds before planting or after harvest and also during fallow periods in between crops in the western US which can further impose selection pressure for evolution of glyphosate resistance.
Importing and introducing herbicide-resistant weed seeds from an infested area to your farm is more common today. Weed-seed contaminated livestock, crop seed, animal feed, hay, manure, soil, and farm equipment all pose potential risks when moving these items between states, counties, farms, and even fields. Use certified crop seed or certified weed-free hay, and fully inspect items that are brought onto the farm to avoid bringing infested inputs onto your farm. Pay particular attention to crop seeds and hay that are grown in regions where problematic resistant weeds are common. For example, herbicide-resistant Palmer amaranth was first discovered on a few farms in Pennsylvania in 2013 and in New York in 2020. But these resistant biotypes had not “evolved” in Pennsylvania or New York; rather, they were imported into these states from the South and Midwest, likely via several different carriers, including livestock feed and farm machinery.
At least seven states have added Palmer amaranth to their Noxious Weed lists to prevent the spread (Figure 6). Without aggressive management, once a resistant biotype is introduced, it can spread throughout the farm, onto neighboring farms, and across the state and the region. With today’s national and global agriculture networks, the potential for introducing new pests to the farm is greater than ever. It is critical to monitor crop and livestock inputs, farm fields, and adjacent areas to ensure you do not import and introduce something that will become a future problem.
Figure 6. Palmer amaranth has been added to Noxious Weed Lists in at least seven states in the last few years including DE, IA, MD, MN, ND, OH, & PA. (Photo credit: Claudio Rubione, GROW)
Herbicides are important tools for weed management, but managing herbicide resistance requires knowing a little more about how active ingredients interact with weeds, as well as other integrated weed management strategies. To help users understand how herbicides control weeds, Herbicide Group Numbers are found on the herbicide labels and containers (See Figure 7). These herbicide group numbers correspond with individual herbicide modes of action (MOA) (see The Basics of Herbicide Resistance for more details). Effective herbicide-resistance management must include rotating herbicide MOA, using multiple effective MOA, and reducing weed exposure to herbicides by integrating multiple management strategies that prevent weed-crop competition and weed seed production. Here is a list of herbicide management considerations to help delay and manage herbicide resistance.
1. Know herbicide MOA group numbers when making herbicide selections, and use multiple effective MOA and rotate MOA within and between growing seasons. Cross resistance within a herbicide MOA is common, so the need to select alternative effective MOA is paramount (See the Take Action Herbicide Classification Chart here).
2. Consider the potential for metabolic resistance in the weeds you are targeting along with the selected herbicide partners (see Sections 8 and 9 in the Basics of Herbicide Resistance).
3. Use full labeled rates with multiple effective herbicides (and MOA) and start clean (plant into a weed-free seedbed).
4. Include effective residual herbicides for soil applications.
5. Include overlapping residual herbicides with postemergence applications. Overlapping herbicides should target the same weeds with different MOA’s.
6. Make timely applications to small annual weeds using appropriate nozzles, spray volume, adjuvants, etc, once weeds are emerged.
7. Monitor fields regularly and scout 7-10 days after postemergence applications to evaluate weed control.
A number of weed species are usually present in any field, and herbicides are typically used to manage both susceptible and resistant biotypes. Herbicide selection depends on a number of factors (see list below) that should include resistance management. It is difficult if not impossible to select multiple effective MOA for every weed species present in a field. However, this tactic is highly recommended for known resistant biotypes and weeds that have high potential to evolve herbicide resistance (see The Basics of Herbicide Resistance for more details). Avoid the repeated use of the same MOA every year and especially multiple times per season. For greater detail about more general considerations for selecting appropriate herbicides, review your state and regional weed control guides (The Mid-Atlantic, Ohio, Indiana, Illinois, and Missouri, Tennessee, Michigan, and Kansas weed control guides are provided as example references in the Citations section of this webpage).
Factors to consider when selecting herbicides in addition to Managing for Herbicide Resistance
Herbicide resistance within or across chemical families with the same mode of action is called cross resistance. For example, cross resistance is common for Group 2 herbicides. Even though there are five different herbicide families within the Group 2 herbicides, they are all ALS inhibitors, so resistance to one herbicide may eliminate the effectiveness of them all (Figure 14).
Figure 14. Cross resistance occurs within or across chemical families with the same mode of action. Pursuit (imazethapyr) and Classic (chlorimuron) belong to different chemical families within the Group 2 herbicides. (Photo credit: Claudio Rubione, GROW; graphic credit: Lourdes Rubione)
Resistance across different modes of action is called multiple resistance. Multiple resistance can occur when resistance evolves after repeated use of a herbicide group followed by the repeated application of other herbicide groups. It seldom occurs simultaneously, although this is less certain with metabolic resistance. The evolution of non-target site resistance complicates both cross and multiple resistance since there is evidence that these can evolve without exposure to specific herbicide groups. This will be discussed more in Section 8.
Here’s an example of how multiple resistance evolves: Let’s say Group 2 and Group 5 herbicides are applied annually for several years for weed control in both corn and soybeans in rotation. Waterhemp control worsens as the waterhemp population first evolves Group 2 and then Group 5 herbicide resistance. In this scenario, the grower then adopts Roundup Ready corn and soybean technology and begins using glyphosate, a Group 9 herbicide, every year in both crops. After multiple years of using glyphosate and the Group 2 and 5 herbicides, the waterhemp population has evolved multiple resistance to three different herbicide groups (2, 5, and 9) (Figure 15).
Figure 15. Multiple resistance is resistance across different modes of action. When a weed is resistant to a Group 2 herbicide, Pursuit (imazethapyr), a Group 5 herbicide, Atrazine, and a Group 9 herbicide, Roundup (glyphosate), it has multiple resistance to three different modes of action. (Photo credit: Claudio Rubione, GROW; graphic credit: Lourdes Rubione)
A recent review in Weed Science on the biochemical and molecular basis for resistance explains that patterns of cross-resistance are often quite complicated and complex. For example, target-site resistance (TSR) to Group 1 herbicides (which will be discussed more in Section 8) is typically due to an amino acid substitution in the ACCase binding site. However, with three different herbicide families belonging to the Group 1 herbicides (FOP’s, DIMs, and DEN) and multiple variations in terms of how and where herbicides bind, resistance to a single active ingredient does not necessarily mean cross resistance to all Group 1 herbicides. For example, in Italian ryegrass, a problematic species with a history of herbicide resistance, some TSR amino acid substitutions confer resistance to all three Group 1 herbicides, while others only impact the FOP’s but not the DIMs or DEN or even only partial resistance or sensitivity to one or more families. As the review paper concludes, resistance to one active ingredient does not always result in resistance across an HRAC group, emphasizing that resistance is much more complex than stating a weed is resistant to a single HRAC group.
Another nuance is that some weedy plants are also more susceptible to resistance evolution. Within the grass family, rigid and Italian ryegrass, barnyardgrass, annual bluegrass, wild oats and goosegrass all have multiple herbicide resistance. But in some other grass species such as annual foxtails (Setaria sp.), multiple resistance is not common. Likewise, Palmer amaranth, waterhemp, smooth and redroot pigweed, horseweed, common and giant ragweed and kochia all have biotypes resistant to multiple modes of action, while other dicot weeds such as eastern black nightshade and Pennsylvania smartweed have not evolved multiple resistance. Several weeds have also evolved both target-site and non-target site resistance. This is discussed more in Section 8.
With few exceptions, resistant and susceptible weeds of the same species look the same (Figure 6). Resistant weeds are often identified by patterns in the field rather than by any differences in appearance. If weeds survive a herbicide application, investigate and rule out other factors affecting herbicide performance before concluding that resistance is responsible.
Good questions to ask are:
The Weed Science Society of America (WSSA) recommends exploring several other factors, including:
Figure 6. Whether herbicide-susceptible or resistant, these individuals look the same. (Photo credit: Claudio Rubione, GROW; graphic credit: Lourdes Rubione)
How do you confirm herbicide resistance? There are three primary ways to confirm herbicide resistance. These include field testing, greenhouse testing and more limited genetic testing for some types of resistance. Once you suspect herbicide resistance, quickly contact your local county Extension agent or ag consultant and seek advice.
Field testing, which involves treating some surviving plants with the same herbicide mode of action, is not very practical, but it may be the first reaction when weeds escape a soil or foliar-applied herbicide that should have been effective. More importantly, if the escaped weed(s) are suspected to be resistant, attempts should be made to prevent them from producing seed and furthering the problem. This will likely involve applying alternative effective herbicide groups or using mechanical control options.
A more accurate method to test for herbicide resistance is through greenhouse confirmation (Figure 7). This requires working closely with your local Extension service and weed science expert to allow some plants to mature for seed collection for a greenhouse assay (test) after the field season ends. Genetic testing for some types of resistance may also be available which can confirm resistance and provide the mechanism(s) at play. Because these types of tests are specialized and limited in availability, they are generally only sought and used for more unique types of resistance.
Plants surviving a herbicide application is not uncommon, since many issues can compromise weed control. Consider several factors when determining if resistance is the cause for lack of control (Figure 8).
Figure 7. Herbicide-susceptible horseweed (left) and resistant horseweed (right) are identified in a greenhouse glyphosate screening test. (Photo credit: Mark VanGessel, University Delaware)
Review the field’s history of weed management practices. What herbicides have been used and how often? What is the cropping and tillage history? What non-chemical weed management tactics are used? What are the problem weeds, and has resistance been identified with these species before?
Know the biology of the escaped weed(s). When did they emerge relative to the herbicide application? For a POST application, what was the age and size of the weedy plants at application?
Examine the environmental conditions before and after herbicide application. Did it rain? What were daytime and nighttime air temperatures? Were the weeds drought stressed? What were the soil characteristics (wet, dry, soil texture, pH, organic matter, etc.)?
Scrutinize the application history. Were there any problems related to equipment, herbicide rate and calibration, herbicide delivery, or wind? Look carefully at the field and the pattern of weed escapes. Are there any identified patterns in the field such as multiple weed species present, or is only a single species present, and should the herbicide(s) control it? Is there little or no injury on the surviving species while other species are controlled? Is the spatial pattern of surviving weeds random or can a sprayer skip or malfunction be identified?
Consider how crop cultural practices may influence the effectiveness of the weed management program. For example, no-till requires a burndown application prior to crop emergence and wider row spacings are less effective at shading weeds potentially allowing weed emergence once the residual herbicide has lost effectiveness.
If you observe multiple weed species escapes, a uniform response of individuals within a population (e.g. all stunted or chlorotic), or a spatial pattern of plants emerging related to the herbicide application, then herbicide resistance is likely NOT the problem. But if you can eliminate potential causes and narrow the list, herbicide resistance may be a factor for poor control and escaped weeds.
Figure 8. These factors can all influence herbicide effectiveness and potential for weed escapes. Be sure to explore all potential causes for reduced weed control before concluding that herbicide resistance is to blame.(Figure adapted from Lesson 4, Scouting After a Herbicide Application and Confirming Herbicide Resistance, WSSA)
After you thoroughly examine the factors that can compromise weed control, this should help answer whether herbicide resistance may indeed be the problem.
How do you confirm herbicide resistance? Greenhouse testing often requires assistance from an institution that has a research greenhouse facility and a great deal of herbicide knowledge. Greenhouse testing often takes a few months to obtain results, particularly if low-level resistance is involved. It requires collecting seeds from mature suspect plants, as well as some seeds of the same species collected from fields where resistance is not a concern (to create a control group). Some knowledge about how to culture the weed species is necessary. The seeds of many species require exposure to a cold (and perhaps moist) environment for up to several months, either after or during the process of maturing, in order to germinate. Some seeds even require “scarification,” a process of physically or chemically treating the seed to create openings in the seed coat to spur germination. Once the plants germinate, emerge, and have developed three or four leaves, they should be sprayed very precisely with several rates of the suspect herbicide (Figure 9). This also requires specialized equipment and trained technical staff. This type of test can confirm or refute resistance, but it does not provide the mechanism of resistance (see Section 8). Most university weed science programs are equipped with specialized equipment and the experience to conduct this type of greenhouse assay. As mentioned previously, you would generally only seek out these services for more unique types of resistance, not for herbicide-resistance cases that are common in your area. Contact your local county Extension service or state Extension weed specialist for more information. Criteria for confirming herbicide resistance according to International Herbicide-Resistant Weed Database can be accessed at: http://weedscience.org/Pages/ResistanceCriterion.pdf.
Figure 9. A greenhouse experiment screening kochia for glyphosate resistance. (Photo credit: Claudio Rubione, GROW)
Genetic testing uses leaves or seeds from the suspected plant. It is very accurate and fast, but it is not widely available in the U.S. The downside of genetic testing is that it only tests for known resistance mechanisms. Consequently, it is possible that a plant could test “negative” for resistance but actually be resistant due to a different mechanism. Because of this, genetic testing is generally used only for common, well-known resistance cases. The University of Illinois Plant Clinic has a molecular assay for glyphosate (Group 9) and PPO (Group 14) resistance in pigweeds. Contact the University of Illinois Plant Clinic for more information. In Canada, several public and private labs across the country test plants and seeds submitted by Canadian farmers or ag professionals for specific types of resistance. In Australia, the Australian Herbicide Resistance Initiative (AHRI) is actively testing both plants and seeds from Australian farms, as is as a lab associated with the University of Adelaide. Other testing opportunities may be available in other American states and in other countries.
There are many ways that weeds evolve resistance to herbicides, but the mechanisms fall into two main categories, 1.) target-site resistance (TSR) and 2.) non-target site resistance (NTSR). TSR occurs when the herbicide can no longer bind to the site of action (Figure 10a), while NTSR occurs when a mechanism prevents lethal or toxic levels of the herbicide from reaching the intended site where the herbicide “acts.” Enhanced metabolism is one of the most concerning types of NTSR and leads to alteration and inactivation of herbicides (Figure 10b. (See Section 8 in the Basics of Herbicide Resistance – What are the mechanisms for herbicide resistance for more details).
Figure 10. Examples of (a) target-site vs. (b) non-target site resistance in plants.
In most cases, it can be difficult to know whether the resistance mechanism at the farm level is TSR or NTSR. Regardless of the mechanism, rotating herbicide modes of action and using multiple effective modes is always important for managing resistance evolution. In addition, effective non-chemical control strategies that emphasize prevention, cultural, mechanical, and biological tactics must be implemented.
Rotating herbicide MOA and using multiple effective herbicide MOA is important for managing resistance evolution. (For more information about herbicide rotation and mixing guidelines, refer to Section 6. that follows). In addition, non-chemical management tactics are not affected by herbicide-resistance mechanisms, so they are critical for long-term weed management.
Target-site resistance has historically been the most common mechanism identified in resistant populations and the most widely studied. This type of resistance occurs when the herbicide can no longer bind to the site of action (Figure 8a). Using herbicides with different effective MOA (tank-mixing, rotating herbicides and using overlapping residuals) is widely promoted to help prevent this dominant type of resistance.
Recently, some research has raised questions about the effectiveness of rotating herbicide MOA in slowing resistance evolution. Australian and Canadian scientists point out that new research suggests herbicide mixtures or sequential applications to the same weed tend to be more effective than herbicide rotation in preventing and managing target-site resistance (Fig. 11). As an example, research conducted at the University of Illinois with glyphosate-resistant waterhemp reported that using multiple effective MOA when tank-mixing was more effective than rotating herbicide MOA in delaying resistance evolution. While these results came from TSR to glyphosate in waterhemp, it is unclear whether these results are applicable to other resistant weeds and herbicides. For herbicide mixing to be most successful, different effective modes of action must be employed at the same time.
Non-target site resistance occurs when a mechanism prevents the herbicide from reaching the intended site where the herbicide “acts” in sufficient lethal quantity. Enhanced metabolism or metabolic resistance is one of the most concerning types of NTSR and is a process within resistant (and tolerant) plants that leads to alteration and inactivation of herbicides (Figure 10b).
Using multiple effective MOA is still a common resistance management recommendation for NTSR, because the probability that a weed will evolve resistance to two different herbicide MOA when used in combination is less likely than the weed evolving resistance to a single herbicide mode. However, the benefit of using multiple effective MOA and rotating herbicide MOA to help manage NTSR resistance is likely limited, because the mechanisms for resistance can be broad-based and cross multiple herbicide groups. As another study conducted at the University of Illinois points out, in many cases, NTSR weed populations may be resistant to multiple MOA and able to survive herbicides from the same and different herbicide groups, including those the weed populations have never yet encountered. In fact, research published in Europe focusing on blackgrass (Alopecurus myosuroides) suggested that while herbicide mixtures reduced the potential for TSR, they might favor the evolution of “generalist resistance mechanisms” such as metabolic resistance.
For mixtures to be effective for resistance management, the Australian team further states they must meet strict standards. Those include providing redundant control of the target weed with multiple herbicide MOA and possessing similar soil persistence or residual activity. These criteria ensure that targeted weeds are always exposed to multiple effective MOA whether they are soil- or foliar-applied.
It is also important to know the multiple resistance status of weed populations in specific fields to inform herbicide use strategies in mixture and in rotation. For example, what is the prevalence of resistance between Group 3 (microtubule inhibitors) and Group 15 (long-chain fatty acid inhibitors) herbicides in rigid ryegrass (Lolium rigidum), the most common weedy annual ryegrass in Australia. Groups 3 and 15 have different MOA and also typically different modes of metabolism in rigid ryegrass. Differing modes of metabolism mean that different enzyme pathways detoxify the herbicides belonging to each group (Cytochrome P450 vs. Glutathione-S-Transferase enzyme families), helping to reduce the potential for multiple NTSR (Figure 12). Identifying these relationships and building herbicide programs based on both herbicide MOA and mode of metabolism or detoxification will be important in the future to prevent the evolution of multiple resistance.
Finally, unfortunately with enhanced metabolism, the potential for cross and multiple resistance without exposure to specific or multiple herbicide groups is a real concern. To prevent metabolic resistance, University of Illinois scientists state that new tactics and technology must be developed that will require a greater emphasis on non-chemical weed management. In addition, they recommend developing predictive tools for herbicide management and rotation recommendations based on multiple-resistance potential. Additional details are available here.
Watch this GROW video from University of Illinois weed scientist Dr. Patrick Tranel for an overview of the dynamics between tank-mixing and herbicide rotation discussed above:
The recommendation to rotate herbicide MOA and use two or more effective MOA in tank mixtures has been a common theme promoted by agricultural professionals to delay resistance. The probability that a weed will evolve resistance to two different herbicide MOA when used in combination is less likely than the weed evolving resistance to a single herbicide MOA. In addition, rotating MOA during and between seasons reduces exposure to the active ingredient and the potential selection pressure it imposes. In the example that follows (Table 1), of the three weeds targeted, only Palmer amaranth is treated with two effective MOA.
Table 1. Introduction to multiple effective modes of action.
Successful use of multiple effective MOA to help manage and delay herbicide resistance depends on the lack of weed population biotypes already resistant to herbicides from certain groups. As an example, in Table 2, without resistance, several effective herbicide MOA can be used to control susceptible waterhemp. However, as multiple resistance becomes more problematic, effective herbicide modes of action become limited. Without resistance, there are herbicides from seven mode of action groups that can target waterhemp. However, if you encounter six-way resistant waterhemp which has been identified in at least two U.S. states, only glufosinate (Group 10) remains to help control the six-way-resistant biotype. The presence of multiple resistance in some of our most problematic weed species has already greatly reduced our ability to use herbicide selection tactics effectively for resistance management.
Table 2. Effectiveness of seven different MOA groups for control of herbicide-susceptible and herbicide-resistant waterhemp in corn or soybean. Waterhemp ranges from susceptible to having six-way herbicide resistance. Six-way multiple-resistant waterhemp has been identified in Missouri, Minnesota, and Illinois and five-way cross resistance in Ontario, Canada, and North Carolina.
Recent research on tank-mixing versus rotating herbicide modes of action has shown new light on which strategy is most important for preventing herbicide resistance, including target-site and non-target-site resistance. For an overview of this, watch this GROW video with University of Illinois weed scientist Dr. Patrick Tranel:
See Beyond Herbicide Site of Action: Considering “Effective” Sites of Action for additional information on strategies for mixing effective herbicide modes of action.
Watch this video here:
There are many ways that weeds evolve resistance to herbicides, but the mechanisms fall into two main categories:
1. Target-site resistance (TSR)
2. Non-target-site resistance (NTSR)
Most plant target sites are enzymes — proteins that function as essential steps in a biological process. They catalyze (initiate) or direct critical reactions necessary for plant growth and development.
Target-site resistance was the first herbicide-resistance mechanism identified and continues to be a very widespread type of resistance. The most common type of TSR occurs when there is a change in the structure of the plant’s target site/protein, so the herbicide can no longer bind to the target site. As a result, the resistant plants survive the herbicide application and continue to grow (Figure 16). Using herbicides with different effective modes of action (by overlapping residuals, tank-mixing, and rotating herbicides) is widely promoted to help prevent this dominant type of resistance. Research conducted at the University of Illinois with waterhemp suggests that using multiple effective modes of action and tank-mixing was more effective than herbicide rotation in delaying TSR evolution.
Non-target site resistance occurs when a process within the plant prevents the herbicide from reaching the intended site where the herbicide “acts.” There are several mechanisms of NTSR including sequestration of the herbicide in the vacuole (a small, enclosed space in a cell) and enhanced metabolism. Enhanced metabolism, also called metabolic resistance, occurs when the herbicide enters the plant cell, is altered chemically and is ultimately rendered immobile, nontoxic, and inactive – in short, unable to do its work in the plant (Figure 17). This is the most concerning type of NTSR, because the mechanism can permit plants to develop resistance to specific or multiple herbicide groups without any physical exposure to them. For example, in 2021, a waterhemp population in Illinois was identified with metabolic resistance to dicamba, a Group 4 herbicide, yet the field had never been treated with dicamba. The dicamba-resistant biotype was also resistant to the Group 4 herbicide, 2,4-D.
Because NTSR and metabolic resistance are more recent discoveries, scientists are still evaluating their implications and looking at potential effective alternatives for managing these kinds of resistance. Unfortunately, NTSR is increasingly common in weed populations.
Figure 16. This is an example of classic target-site resistance with the Group 5 herbicides (Photosystem II inhibitors). The herbicide is (a) applied to the weed (common ragweed here), (b) enters the plant cell and (c) the chloroplast and (d) binds to a key protein that stops photosynthesis. With target-site resistance, the herbicide can no longer bind to the target site (d) in the resistant biotype, and photosynthesis continues. (Illustration adapted by William Curran from University of Minnesota Extension; Graphic credit: Lourdes Rubione)
Figure 17. Typically, in the first step in herbicide metabolism, the herbicide is altered and sometimes inactivated. In this example, an enzyme alters 2,4-D by cleaving (removing) a chloride ion and replacing it with an hydroxyl (OH). The herbicide is further metabolized by other enzymes and eventually either stored or incorporated into various plant parts. (Image credit: William Curran, Penn State University; Graphic credit: Lourdes Rubione)
Watch this GROW video from University of Illinois weed scientist Dr. Patrick Tranel, for an overview of the differences between target-site and non-target-site resistance:
In addition to a change in the structure of the target-site enzyme/protein, another type of target-site resistance is due to increased production of the target-site protein. This occurs when there is an increase in the expression of the target site/protein, or there are increased copies of the gene that codes for the target site/protein (amplification) (Figure 18). These are less common and often associated with low-level resistance (discussed at the end of this section). This type of resistance has been identified most commonly for glyphosate in several weeds including rigid ryegrass, horseweed, and Palmer amaranth.
Figure 18. Another mechanism for target site resistance is an increase in the expression of the target site/protein or in the copies of the gene that codes for the target site/protein (amplification). The plant produces higher amounts of the target-site protein overwhelming the ability of the herbicide to completely disrupt some physiological process. (Image credit: William Curran, Penn State University; Graphic credit: Lourdes Rubione)
Mechanisms of non-target site resistance include:
Decreased translocation and sequestration have been reported with several herbicides and weeds. This occurs when less of the herbicide reaches the target site. Some of the best known examples include rigid ryegrass with glyphosate and Italian ryegrass and horseweed with paraquat. Certain populations of giant ragweed evolved the ability to rapidly desiccate treated leaves, trapping the glyphosate and preventing translocation to the site of action. This has been described as the “Phoenix phenomenon” or “Phoenix resistance” (Figure 19).
Reduced foliar uptake or absorption of the herbicide is an uncommon NTSR mechanism that has been reported with several herbicides and weeds including waterhemp with glyphosate and prickly lettuce with 2,4-D. A common suspect is the production of greater amounts of cuticular wax on plant leaves, which could reduce plant uptake, but this mechanism has not always been confirmed.
Figure 19. The “Phoenix phenomenon” in giant ragweed treated with glyphosate is shown above. Compare (a) a glyphosate-susceptible plant at 2 days and (b) 21 days after treatment, with (c) a glyphosate-resistant plant at 2 days and (d) 21 days after treatment. With the Phoenix phenomenon, the older leaves desiccate rapidly, trapping the glyphosate in the dead tissue, while the new shoots continue to grow. (Image credit: Van Horn et al. 2018)
What is enhanced metabolism, or metabolic resistance? Plants have mechanisms to deal with all sorts of potentially harmful compounds in the environment. Herbicides are just one type of compound that some plants have the ability to detoxify. Metabolic resistance is a process within resistant (and tolerant) plants that leads to alteration and inactivation of herbicides.
With metabolic resistance, once the herbicide enters the plant cell, it is altered chemically to form one or more metabolites. Metabolites are often bound to plant structures such as amino acids, proteins, lignin, or sugars to form conjugates. Once bound or conjugated, herbicides are usually rendered immobile, nontoxic, and inactive – unable to do their work in the plant. What is most alarming is the mechanisms for metabolic resistance can be broad-based and cross multiple herbicide modes of action.
Metabolic resistance is often described in three phases (Figure 20). In the first phase, an enzyme such as Cytochrome P450 removes/cleaves off a sidechain from the herbicide molecule, sometimes inactivating the herbicide. In the example illustration with 2,4-D, a chloride atom is removed and replaced by a hydroxyl group. This is usually an oxidation reaction. In phase two, the hydroxyl group is very reactive and quickly removed and the herbicide molecule joins glucose, or sometimes an amino acid, to form a conjugate. In our example, 2,4-D reacts with glucose. In phase 3, the new conjugate may be transported to a vacuole and stored or may continue to join larger molecules, forming secondary conjugates and eventually helping to build starch or cellulose.
Figure 20. See the three phases of herbicide metabolism, using 2,4-D as an example, above. (Image credit: William Curran; Graphic credit: Lourdes Rubione)
These metabolic processes usually have an impact across a broad range of chemicals and may be capable of inactivating herbicide compounds that the plant has never encountered. Rigid ryegrass (Lolium rigidum) in Australia and other Lolium species may be the best examples of weeds with both TSR and NTSR and many cases of cross and multiple resistance. A biotype of Lolium rigidum in Australia has evolved resistance to seven different modes of action displaying a variety of mechanisms. Metabolic resistance in Lolium can be responsible for both the resistance to Group 1 and 2 herbicides as well as Groups 5 and 6. Reduced glyphosate translocation in Lolium spp. has also been identified as a NTSR mechanism in a number of countries.
Unlike target-site, metabolic resistance could quickly result in broad cross-resistance, potentially eliminating the effectiveness of herbicides from multiple modes of action. Metabolic resistance is quickly gaining ground with several herbicide groups including 1, 2, 4, 5, 9, 14, 15, and 27. Previously recommended herbicide management approaches, such as tank-mixing modes of action or rotating herbicides, are not always effective against metabolic resistance. New approaches must be developed that will require a greater emphasis on non-chemical weed management.
Some herbicides have demonstrated both TSR and NTSR in some weeds. Groups 1, 2, 4, 5, 9, and 14 have shown both TSR and NTSR in some weed species. The herbicide glyphosate (Group 9), one of the most studied cases of resistance, has demonstrated TSR as an altered EPSPS enzyme, as well as amplification of the gene encoding the EPSPS enzyme. NTSR mechanisms for glyphosate include sequestration in the vacuole and enhanced metabolism.
High-level vs. low-level resistance. The response of individual plants can be different based on the herbicide, weed species, and mechanism(s) for resistance. Plants with high-level resistance may tolerate a 100X dose or more of the herbicide (Figure 21). Target-site resistance and a change in the structure of the target protein is usually responsible for high-level resistance. High-level resistance has been identified in Groups 1, 2, and 5.
Low-level resistance may express itself with a range of plant responses, from slight injury to nearly fatal injury. With low-level resistance, a 2X or 3X dose may still kill the resistant biotype. This type of resistance can result from both TSR and NTSR. Low-level resistance is more common in Groups 4, 9, 14, and 22. Historically, low-level resistance has taken longer to detect since it mimics many responses that weeds have when treated with herbicides under less-than-ideal conditions. (See Section 5).
Sidenote: Some of the latest herbicide-resistant crop technologies were actually developed using metabolic resistance. Enlist Corn is a new multiple herbicide-resistant corn trait that confers resistance to 2,4-D, glyphosate, and the Group 1 aryloxyphenoxypropionate herbicide family (FOP herbicides). The resistant corn can metabolize both the Group 4 herbicide 2,4-D and the Group 1 FOP herbicides because of their similar chemical structure. Glyphosate resistance in Enlist corn is based on target-site resistance.
Figure 21. This waterhemp population with high-level resistance to atrazine is able to survive a dose of 50 lb/acre, whereas a normal sensitive population was controlled at 0.5 lb/acre. A typical field use rate of atrazine is 1.5 lb/acre. (Photo credit: Patrick. Tranel, University of Illinois)
Figure 3. Scouting is a key step in the prevention of herbicide resistance.
A number of approaches should be used when managing herbicide resistance. Prevention and control are key and aim to head off the introduction of resistant weeds in a field or reduce an already established problem. Monitoring crop fields and weed populations by regularly scouting should be implemented to help guide decisions (Figure 2).
Preventative tactics for herbicide-resistant weeds should focus on avoiding weed seed introduction from contaminated crop seed, soil, manure, compost, hay, livestock feed, machinery, neighboring fields and field edges (Figure 3). In addition to seeds from annual and biennial species, the vegetative structures such as rhizomes, stolons, tubers, and bulbs of some perennials can also help spread problem weed infestations. In addition, prevention should go much further and include tactics that also slow the resistance evolution process by reducing weed exposure to herbicides within the same MOA group and integrating multiple management strategies that prevent selection and spread. See this GROW webpage for detailed information on prevention.
Figure 3. Weed seeds can spread across the farm many ways.
IWM is essential to help prevent yield and quality loss, halt the introduction of more weed seed, and reduce the problem over time. Cultural, chemical, mechanical, and sometimes biological weed control tactics are critical for maintaining successful and profitable crop production (Figure 4). If you discover a new problematic weed on the farm that can still be contained, aggressively manage the infestation to control the population and prevent seed production. As an example, if Palmer amaranth was recently introduced and only a few dozen plants are identified in a field, remove them by hand to prevent the weeds from reproducing and spreading. If the plants are flowering, carefully remove and bag the entire plant being careful not to spread seeds. For these small infestations, burn or bury plants outside the field deep enough to prevent survival. If a resistant weed population has become more widely established on the farm, consider all appropriate options, based on the characteristics of the species, to drive down the weed infestation over time and maintain successful and profitable crop production (Figure 4).
Figure 4. Examples of IWM practices to manage herbicide-resistant weeds. (Photo credits: Claudio Rubione, GROW)
GROW’s Farmer Case Studies page contains video interviews with farmers across the country who are experimenting with or have mastered the use of multiple integrated weed management tactics to control weeds on their operations. Visit the webpage to learn more.
Non-chemical strategies include prevention tactics, cultural production practices, mechanical systems, and biological tools. Limiting sunlight from reaching a weed is one of the most basic but important tools for weed control. To accomplish this:
1. Use cultural production practices that encourage the development of a quick, dense crop canopy or cover crop stand to help shade and suppress weeds. See this GROW Cultural Production Practices webpage for additional information on production practices that can aid weed management.
2. Add crops with different planting dates to your rotation, particularly crops that have different life cycles (i.e. fall-seeded, early-spring seeded, summer seeded).
3. If possible, selectively include tillage when you can target specific weed problems such as perennials or by burying the seeds of summer annuals.
4. Be sure to control weed escapes prior to seed set to reduce future weed populations and prevent resistant weeds from spreading. This may require walking fields and using hand removal which was common before the introduction of herbicide-resistant crops. If seed heads are present, remove plants by hand, bag them, and dispose of them outside the crop field by deep burial or burning. For larger infestations, consider adopting some newer technology such as weed electrocution and inter-row mowing (https://growiwm.org/mowing-zapping-and-outcompeting-weeds-opportunities-and-tradeoffs/) as well as harvest weed seed control tactics that include chaff lining or using seed impact mills.
5. Reduce the introduction of weed seed into crop fields by managing field edges with mowing or spot spraying and clean equipment between problematic fields and clean fields, so you don’t spread the problem.
6. Harvest problem fields or weedy areas last to prevent the spread into other fields.
7. Clean farm equipment, including the combine, regularly to reduce seed spread.
For more information, visit the GROW Weed Management Toolbox, and see Section 4 in the Basics of Herbicide Resistance.
Integrated weed management (IWM) is a system that layers multiple weed control methods to suppress and manage weeds over time, by targeting weeds in diverse ways and at various stages of development. IWM requires expanding your weed management practices over more than one season. This requires a better understanding of the weed species present in the field and their biology, such as lifecycle, emergence timing, growth rate, and seed production. The result is a robust weed management program that doesn’t rely on a single herbicide mode of action or any other sole management tactic. In general, IWM techniques include Prevention, Cultural, Chemical, Mechanical, and Biological tactics (Figure 1). See more details on these GROW webpages on what IWM is and how you can combine weed control tactics effectively.
Herbicide-resistant individuals initially may be present in a weed population at exceptionally low frequency. These “biotypes” differ from the majority population because they have mechanism(s) that allows them to survive exposure to the herbicide. Maybe only one individual out of 10 million plants carries the resistant trait. Often the frequency or rate at which this mutation occurs within a weed species is much lower than this. But, with repeated application of the same herbicide or herbicide group over time, without the use of other effective weed control strategies, the frequency of the resistant individuals or biotype within a population increases (Figure 3). Although the herbicide is still controlling susceptible individuals, the resistant biotype survives and produces seed. Using multiple effective modes of action (MOA), rotating modes of action and using non-chemical control tactics can help reduce selection for herbicide-resistant weeds.
Figure 3. After repeated use of the same herbicide group for multiple seasons, a resistant biotype may be selected that survives the application. As illustrated, the resistant individual (a) survives the herbicide and can (b) mature and produce seed. If the herbicide program continues, (c) more individuals survive the herbicide, they mature and produce seed and eventually, (d) the dominant biotype in the field is resistant. (Adapted by William Curran and Lourdes Rubione from University of Minnesota’s herbicide-resistance management webpage).
Depending on the weed species and the growing conditions, plants can produce anywhere from a few hundred seeds to more than 100,000 seeds per plant for species like pigweeds. The soil in a typical agricultural field contains hundreds to several thousand seeds per square foot. One thousand seeds per square foot equates to over 43 million seeds per acre. At initial low frequency, resistant plants can be hard to detect as they evolve and survive in a field. Figure 4 shows how resistant Palmer amaranth quickly spread through a field within three growing seasons when it was not effectively managed.
In addition to herbicides, several factors can reduce weed survival, such as environmental conditions, plant pathogens, and predation. Even when herbicides are 90% or more effective, some weed escapes are expected. Once the percentage of a resistant weed species population reaches about 25%, it becomes evident from the escaped weeds that something has changed. Once resistance appears, it can spread quickly and unfortunately, a gradual decline in performance over time is rarely observed. Rather, unless the field is being scouted regularly, the shift to a dominant herbicide-resistant weed biotype may be missed until poor weed control becomes very visible.
Herbicide resistance can evolve quickly over time with frequent use of the same herbicide mode of action and without use of other effective weed control strategies. Resistance from some herbicide groups was evident after three to five years of repeated use, but with other modes of action, it may take seven to 10 (or more) years before pervasive resistance becomes evident. The herbicide mode of action and application frequency, specific weeds and their genetic potential for resistance, and the diversity of weed management tactics practiced on the farm all influence this timeline.
We can observe a similar shift in species/biotypes with other agricultural practices and even in other managed ecosystems. For example, management practices can shift a weed or plant population away from some species/biotypes and towards others, when other effective weed control tactics are not used.
Examples of weed species’ shifts from non-chemical weed management:
Figure 4. These images illustrate how herbicide-resistant Palmer amaranth rapidly escapes control over time, from an escaped plant here and there early in the infestation (1st year), to a more visible spread of herbicide-resistant Palmer amaranth (2nd year), to domination of the field by the herbicide-resistant biotype (3rd year). (Photo credits: Art Bradley and Alan York, NC State University)
Some herbicide mode of action groups are more prone to develop herbicide resistance from frequent use. Weeds emerged with resistance to Group 2 herbicides in the early- to mid-1980s, only a few years after their introduction (Figure 22). Since that time, more than 170 weed species have been identified with Group 2 resistance. The second most problematic herbicide mode of action is the Group 5 herbicides, although much of this resistance developed back in the 1970s and 1980s with atrazine use in corn (Figure 22). Glyphosate, a Group 9 herbicide, became the third most problematic mode of action, but only after the widespread and frequent use of glyphosate in Roundup Ready crops starting in the late 1990s accelerated resistance. The ACCase inhibitors or Group 1 herbicides are in fourth place, based on the number of resistant weed species, followed by the Group 4 synthetic auxins (Figure 22).
Figure 22. This chart shows the number of resistant species for several herbicide modes of action. These are the top five herbicide MOA’s based on the number of resistant weed species. (Chart credit: The International Herbicide-Resistant Weed Database, accessed online, May 20, 2024)
Some weedy plants are also more susceptible to resistance evolution (Figure 23). Within the grass family (Poaceae), rigid and Italian ryegrass, barnyardgrass, annual bluegrass, wild oats and goosegrass all have multiple resistance (Figure 24). Palmer amaranth, waterhemp, smooth and redroot pigweed, horseweed, and both common and giant ragweed all have biotypes resistant to multiple modes of action. Several weeds have evolved both target-site resistance and non-target-site resistance. The problem species discussed most often in both the scientific and ag communities include rigid ryegrass in Australia and Palmer amaranth and waterhemp in the U.S.
Figure 23. This graph shows the number of herbicide-resistant weed species by plant family. (Chart credit: The International Herbicide-Resistant Weed Database, accessed online, May 20, 2024)
Figure 24. This graph displays the top weed species resistant to multiple herbicide modes of action. (Chart credit: The International Herbicide-Resistant Weed Database, accessed online, May 20, 2024)
In general, herbicides are at higher risk for resistance if they target a single site of action, and if the weed species have a higher frequency of the resistant biotype in the population (e.g. one in one million vs. one in 10 million individuals carry the resistance trait) or if the target field has a greater population size (e.g. 100 seeds vs 1,000 seeds per square foot in the soil seed bank). Resistance evolution takes longer for some herbicides and weeds, especially if the herbicide targets multiple sites of action, is used in combination with other herbicides, and is used with other effective non-chemical weed control options.
Weeds with diverse genetic backgrounds are more prone to resistance evolution. The annual ryegrasses and some pigweeds both have diverse genetic backgrounds. Two of the most important characteristics for evolution of resistance are reproductive capability and how seeds disperse and spread into new areas. Some of the most problematic weeds produce thousands of seeds, the seeds are wind dispersed and/or the plants require outcrossing for successful reproduction. For example, waterhemp and Palmer amaranth can produce over 100,000 seeds per plant, have separate male and female plants (dioecious), and resistance moves with wind-blown pollen. The herbicide mode of action and application frequency, targeted weed species and genetic potential for resistance, and the diversity of weed management tactics all influence the potential for resistance evolution.
Once you have herbicide-resistant biotypes in your fields, it is unlikely that they will revert to susceptible biotypes within a farming generation, even if you stop using the herbicides that they resist. This is especially true if the resistant biotype becomes established for several years and is producing seed annually. Once resistance is established, fields typically will have a mixture of susceptible and resistant biotypes (we’ll refer to them as S and R biotypes). The ratio of these individuals could change over time depending on herbicide use. With repeated use of the same herbicide group over time, the population of a resistant biotype will increase and be the dominant biotype.
There is a phenomenon in plants known as a “fitness penalty,” wherein the resistance trait comes with inferior growth and/or reproduction. But in most instances, herbicide resistance does not appear to introduce a substantial fitness penalty. As a result, even if the problem herbicide group is no longer used, and a portion of the susceptible seedbank remains, the population will likely continue to be a mixture of R and S biotypes. Depending on the species and other management inputs, weed seeds can persist in the soil for several years. Seeds buried deeper in the plow layer tend to remain viable longer than seeds closer to the soil surface. Of course, seeds buried below the depth they are capable of emerging pose little risk, unless they are brought back near the soil surface with tillage. The seeds of some species, such as giant foxtail and other annual grasses, generally survive for only a few years. Others with harder seed coats, such as common lambsquarters and velvetleaf, might survive for decades in the soil under the right conditions. Depending on the weed species, this could impact the longevity of both R and S biotype seeds in the soil. If there are no weed seeds produced at the end of the growing season, the size of the weed seed bank will decline as seeds die, and the frequency of R and S biotypes should remain the same.
A herbicide mode of action describes the way a herbicide works to control weeds. In general, herbicides interrupt certain biological processes, often by disrupting enzyme activity or other plant functions. The numerical mode of action system groups herbicides and provides a reference for growers and ag professionals to rotate modes of action and help design integrated weed management programs (Figure 10). Currently, herbicides are grouped into 25 different modes of action (plus an additional group of herbicides with unknown modes of action for 26 total groups). Each class is identified by a Group Number.
The herbicide site of action, or target site, is the location of the primary interruption/disruption and is often a specific plant enzyme. Enzymes are proteins that function like stairsteps in a biological process required for plant growth. In most cases, herbicides within the same mode of action group have the same site of action. For example, all Group 1 herbicides inhibit the enzyme acetyl CoA carboxylase (ACCase inhibitors) and all the Group 2 herbicides inhibit the enzyme acetolactate synthase (ALS inhibitors) (Figure 10).
Figure 10. Take Action Herbicide Classification by Mode of Action Poster. In this chart, herbicides are grouped by mode of action. This visible portion shows three groups (1, 2, and 9), the chemical family, active ingredient, and branded product examples. Premix products and their active ingredients and trade names are also shown.
The herbicide mode of action describes the way a herbicide works to control weeds by interrupting a certain biological process. Herbicides with the same mode of action share a Group Number and have similar properties (Figure 10). For example, ACCase inhibitors are the mode of action of the postemergence grass-specific herbicides and includes three different chemical families. All three families have the same mode of action and belong to Group 1. Clethodim and quizalofop are example Group 1 active ingredients. The Group 2 herbicides are the ALS inhibitors and include five different chemical families. Imazamox and chlorimuron are example Group 2 active ingredients. Glyphosate is a Group 9 herbicide. The Group 9 mode of action is the inhibition of EPSP synthase. Of the 26 different herbicide groups, 17 are commonly used in U.S. agriculture. For ag professionals and farmers, knowing the Group Number classification is important for using multiple effective modes of action and for herbicide rotation.
The chemical family is based on the chemical structure of the herbicide molecule. There are three chemical families in the Group 1 herbicides including the aryloxyphenoxypropionate (FOP), cyclohexanedione (DIM), and phenylpyrazolin (DEN) (Figure 10). The active ingredient, also known as the common name for the herbicide, is listed on the herbicide label. Examples of Group 1 common names include quizalofop, clethodim, and pinoxaden. Finally, labels also list herbicide trade names, the brand names the herbicides are sold under. For example, herbicide trade names include Assure II (quizalofop), Select (clethodim), and Axial XL (pinoxaden). Many herbicide products contain more than one herbicide and are sold as prepackaged mixtures (premixes). Product labels contain the herbicide group number for all the active ingredients. Axial Star is a premix of pinoxaden (Group 1) and fluroxypyr (Group 4) (Figure 11).
Figure 11. This herbicide label cover page displays the herbicide group numbers as well as active ingredients. Axial Star contains herbicide active ingredients with two different modes of action and thus, two different group numbers. (Image credit: https://www.syngenta-us.com/current-label/axial_star, accessed online, Nov. 2, 2023)
The global Herbicide Resistance Action committee, (HRAC) is an international body founded by the agrochemical industry, which has developed the most current mode of action classification scheme with corresponding group numbers. The 25 known herbicide modes of action fall into three broad categories based on their mode of action: light activation, cellular metabolism, and cell division and growth. HRAC has published a MAP in poster form that shows how the different herbicide modes of actions are grouped (Figure 12). Herbicide groups target different physiological processes in the plant cell based on mode of action. These processes can include photosynthesis, fatty acid synthesis, amino acid synthesis, and other processes (Figure 13).
Figure 12. The HRAC Mode of Action Classification Map provides more detail on herbicide mode of action and the herbicide target site in the plant cell. (Image credit: HRAC)
Figure 13. This illustration shows the target of different herbicide groups in the plant cell based on mode of action. Herbicides disrupt key physiological processes within the plant cells, including photosynthesis, fatty acid synthesis, amino acid synthesis, and other processes. (Illustration adapted by Lourdes Rubione and William Curran from Delye et al. 2013 Trends in Genetics)
Discovering a new herbicide mode of action is a priority in both the private and public sectors. Some of the major herbicide manufacturers have increased efforts to discover new herbicide molecules. Both private and public institutions are looking for naturally occurring or novel compounds that could be developed into effective commercial herbicides (Figure 25). Several startup companies are also investing in the search. Newer herbicide discovery technologies should help with these efforts, including artificial intelligence, X-ray crystallography, and the use of DNA-encoded libraries.
One of the biggest differences in today’s search compared to past efforts is that companies now investigate the mode of action early in the discovery process. Companies identify critical new enzymes and search for molecules that inhibit them, thus targeting enzymes that have not been targeted previously.
If a new effective herbicide mode of action comes to the marketplace, what’s the chance for resistance evolution? Assuming the new mode of action targets a protein/enzyme that is an essential critical pathway for plant growth and development, the potential for resistance evolution remains high, if – as in the past – the new product is widely adopted and frequently used. More novel chemical solutions that do not rely on targeting single pathways in plants may have greater longevity, but they are much more difficult to discover and develop. The bottom line is that farmers will need to use a wider variety of weed management techniques now and into the future to prevent and manage herbicide-resistant weeds.
Figure 25. Herbicide discovery efforts seeking out new modes of action, such as in vitro assays to screen herbicide modes of action (middle), are on the rise from both major herbicide manufacturers and some newer startups, as well as the public sector. Private sector research and development teams include scientists and associates guiding robust discovery and development pipelines. (Photo credits: Top: Csail.mit.edu News, Feb. 20, 2020; Middle: Plant and Soil Sciences eLibrary, University of Nebraska, Lincoln; Bottom: https://www.fmc.com/en/innovation/research-development).
The future of discovering new and novel herbicides and other chemical solutions has many challenges, but also holds promise. Natural products are the byproducts of microorganisms or plant extracts. Very few of the world’s potential natural products have been screened for herbicidal activity, and several plant extracts show promise as cost-effective commercial herbicides. Unfortunately, very few bioherbicides have been commercialized as production and formulation challenges continued to complicate their success. However, in the effort to develop these compounds, scientists can sometimes discover new herbicide target sites. For example, glufosinate, a Group 10 herbicide discovered back in the 1970s, was developed based on phosphinothricin, a byproduct produced by Streptomyces bacteria.
RNA interference (RNAi) technology is under investigation as a tool to silence key plant genes leading to either enhanced susceptibility to herbicides or outright control of resistant weeds (Westwood et al. 2018). Most of this research has studied RNAi herbicides applied as a spray to target a specific weed species or groups of related species. In addition to weed management, researchers are developing and studying RNAi technology to manage insect pests and plant pathogens, as well as treat human disease. Challenges include survival in the environment, formulation, large-scale production, and product registration.
Synergists, chemical additives that enhance the effectiveness of a chemical, are also an area of interest for researchers looking to combat metabolic resistance. The presence of metabolic resistance eliminates the effectiveness of several current herbicide groups and could render a new mode of action ineffective before it even reaches the marketplace. The idea of adding a synergist to the herbicide spray to counteract the herbicide-degrading enzymes (such as cytochrome P450s or glutathione-S-transferases) is appealing. However, as Shaner and Beckie point out in a 2014 paper, one of the biggest challenges in using this approach is avoiding harming the crop, in addition to the weeds.
New herbicide safeners could be discovered that allow greater selectivity between crops and weeds.
The development of new herbicide-resistant crop technologies could also provide some relief. Some of this discussion centers on developing new, nonselective or broad-spectrum herbicides with novel modes of action not prone to resistance in conjunction with crops that have been engineered to resist the herbicides.
Finally, new herbicide selection predictive tools based on the potential for cross-resistance may be developed. Using these tools to select different herbicide modes of action that will reduce the potential for non-target-site or metabolic resistance would be a major step forward in herbicide resistance management.
Herbicide resistance is the naturally occurring, inheritable ability of an individual plant or population to survive a herbicide application that would kill a normal plant or population of the same species. These surviving plants can mature and produce seed, and the resistance trait is passed on to the next generation. Herbicide susceptibility means the weed or crop are killed by the normal use rate of the herbicide; as an example, the foxtails and other annual grasses are susceptible to the Group 15 herbicides such as s-metolachlor. In contrast, herbicide tolerance is the inherent ability of an entire plant species to survive and reproduce after herbicide treatment at a normal use rate. This term is generally used to describe both weedy plants and crops that were never susceptible to certain herbicides. For example, many broadleaf weeds are susceptible to the group 4 synthetic auxin herbicides, while grassy weeds like giant foxtail and johnsongrass are naturally tolerant. Most grassy weeds are susceptible to the group 1 grass-specific herbicides, while all broadleaf or dicot weeds are tolerant to those herbicides.
Figure 1. Examples of key herbicide-resistant weed species across the U.S.
Resistance in a plant may be naturally occurring or it may be introduced through genetic engineering or other techniques. By definition, crops that have been developed to withstand herbicides that previously would kill them (e.g. the Roundup Ready trait) are herbicide-resistant crops. Herbicide-tolerant crops have the natural, inherent ability to survive the herbicide.
The level of herbicide resistance in weeds can vary due to weed biology as well as the resistance mechanism. A weed with low-level resistance might only survive the labeled herbicide rate, while some species with high-level resistance might survive a dose 100X the labeled rate or greater. A population of common ragweed in Delaware is resistant to the group 2 herbicide cloransulam (FirstRate) and can survive at least a 25X dose (Figure 2). Some of these differences are due to the type of resistance that evolved, which can include target-site and non-target-site. Read more about these resistance types under Section 8.
Figure 2. Two populations of common ragweed sprayed with three rates of cloransulam (FirstRate). The top row is the resistant population and the bottom row is the susceptible population. From left to right are untreated plants, and those treated with cloransulam at 1X, 5X, and 25X a normal field use rate. (Photo credit: Mark VanGessel, University of Delaware)